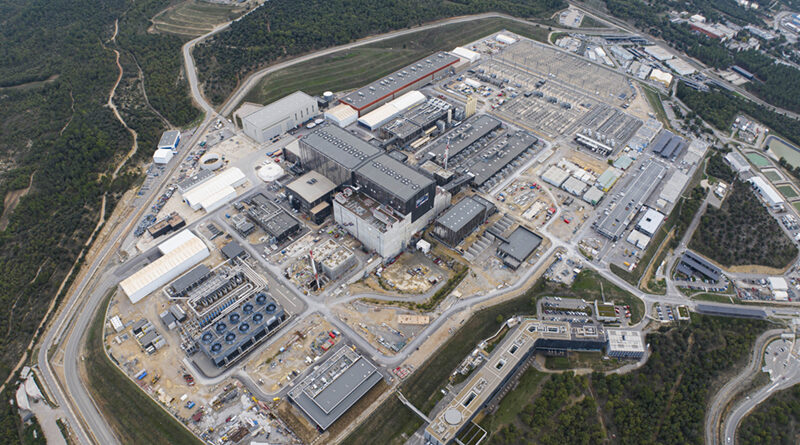
The potential of nuclear fusion as a sustainable solution for global energy security
The quest to demonstrate fusion energy as a practical source of power has occupied scientists and engineers for more than six decades. The longevity and persistence of this effort reflects both the great potential of fusion, and the great challenges in bringing it to reality. The emergence of private sector investment in fusion projects in recent years is a welcome development, complementary to public sector research; but it remains difficult to predict with precision when fusion will be ready to contribute meaningfully to the global energy supply.
Fusion’s potential is tied to its predicted advantages. As with nuclear fission, nuclear fusion is expected to provide baseload power with minimal to no carbon emissions. The energy released by the fusion reaction is intense, several times stronger than the energy released from fission.
Fusion also offers safety advantages: unlike fission, fusion does not rely on a chain reaction, nor does it build up decay heat, so the physics of fusion cannot lead to a Chernobyl-style core meltdown. The quantities of radioactive waste from fusion are likely to be a small fraction of the waste from fission, with much shorter half-lives. And the fuel for fusion is viewed as abundant: deuterium can be extracted from water, including seawater; and tritium, while scarce in itself, can be bred from lithium, which is abundant in the earth’s crust.
But within each of these “advantages” also lies another challenge, a flip side of the coin. While safety is an advantage, it also means that sustaining the fusion reaction for long periods is difficult, requiring sophisticated control systems, careful choice of the materials that house the fusion reaction, and avoidance of plasma disruptions. Achieving “steady-state” operations at high efficiency has yet to be demonstrated by any fusion device. The recent breakthroughs by China’s Experimental Advanced Superconducting Tokamak (EAST) and France’s Tungsten Environment in Steady-state Tokamak (WEST), involving plasma pulses lasting more than 20 minutes, are noteworthy achievements; but in fusion terms, these were relatively low energy plasmas, not involving deuterium-tritium fusion.
In other words: the global fusion community is making progress, in both the public and private sector, but the end goal remains elusive. When I am asked “When will fusion be commercially available?”—a common question from journalists and politicians alike—I answer honestly: “I don’t know.”
ITER, a collaboration of 35 countries located in the south of France and the largest nuclear fusion project in the world, is designed to achieve and sustain fusion reactions at a scale that will enable the study of a controlled “burning” (largely self-heating) plasma. ITER is designed to achieve 400-second pulses of deuterium-tritium fusion with a thermal output of 500 megawatts—and to achieve “steady-state” operations at somewhat lower energy.
But achieving sustained fusion reactions is only one piece of the puzzle. ITER will contribute to addressing several other key remaining challenges, in an integrated manner; and further R&D will be needed to deliver a credible design for a next-phase demonstration reactor.
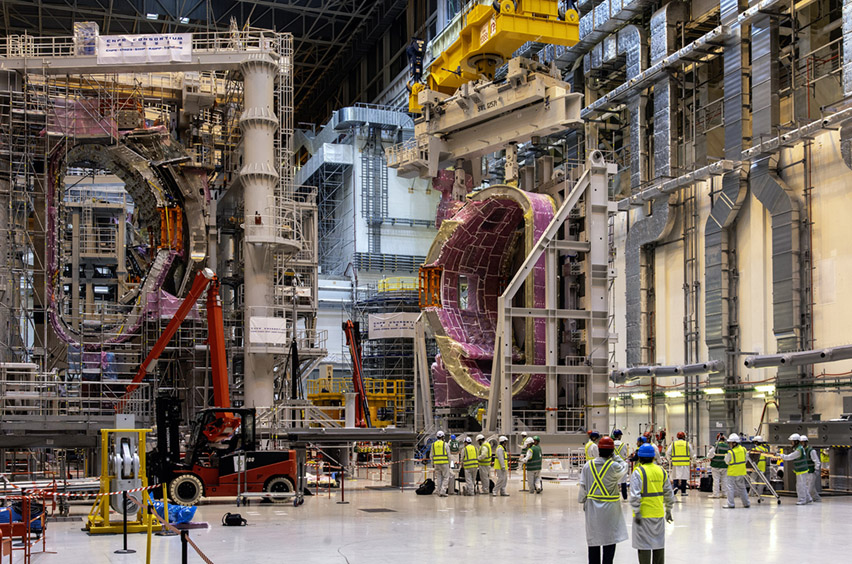
Materials resistant to extreme conditions: The extremely high energy of the neutron coming from the nuclear fusion reaction is both an advantage and a challenge. The intense flux of these high-energy neutrons and other particles generated during fusion reactions subject the structural materials of the fusion device—in ITER’s case, a tokamak—to extreme conditions. Identifying materials capable of withstanding these conditions while maintaining structural integrity is a top priority across the fusion research community. These materials must be compatible with enabling plasma purity—minimizing plasma interactions with the “first wall” surrounding the fusion plasma. They must also be able to withstand the extreme thermal, radiation and vacuum environments inside the reactor without requiring frequent costly replacement. And since the walls of the tokamak become radioactive from bombardment by fusion neutrons, the material must be chosen carefully to achieve the desired goal of not generating long-lived radioactive waste.
ITER has chosen tungsten as the best-suited first wall material available today; but more materials science R&D is needed to identify materials that can withstand the rigors of long-term fusion operation. Could the use of AI accelerate this search? Time will tell.
Robotics for maintenance: The harsh environments generated by nuclear fusion operations make these reactors inaccessible to human operators. Maintenance and repair tasks must thus be performed remotely using advanced robotic systems. ITER is developing state-of-the-art remote handling technologies to address the challenge, but ensuring the reliability and efficiency of these systems in a fusion environment remains a significant engineering hurdle. Current research is focused on enhancing the dexterity, agility and autonomy of remote handling systems to enable efficient maintenance operations in future fusion power plants and to optimize the size of associated maintenance facilities.
Tritium fuel cycle: Tritium, an isotope of hydrogen, is a key fuel for deuterium-tritium (D-T) fusion reactors. However, tritium is scarce in nature; for use in fusion, it must be produced artificially. Additionally, tritium has a relatively short (12.3 year) half-life. While tritium breeding has been demonstrated at small scales, the development of efficient techniques for large-scale, sustained tritium breeding—as well as for extracting and processing the tritium for re-use as fuel—is essential to make a D-T fusion reactor practical. ITER will conduct experiments to test tritium breeding blankets and fuel cycle technologies, but further research is needed to demonstrate the feasibility of industrial tritium production and recycling.
Heat exhaust management in the divertor region: Another primary challenge in fusion reactor design is managing the heat and particle exhaust from the plasma. In tokamaks like ITER, this is accomplished through a component called the divertor, which extracts heat and particles from the plasma and protects the tokamak walls from damage. The extreme power density conditions in the divertor region present significant engineering challenges. ITER is pioneering novel divertor designs and cooling techniques at the present limits of technology. Ongoing research is needed to optimize heat exhaust management in future reactors.
Efficient heat removal for electricity generation: While nuclear fusion produces intense energy, the physical structure of tokamak fusion reactors is that of a geometric heat source, poorly configured to efficiently remove the heat produced from the fusion reaction. Traditional heat removal designs used in fossil fuel or nuclear fission plants interface directly with the heat generation process—often with the water flowing directly past the fuel, both as a moderator of the reaction (in the case of fission), and as an efficient transport mechanism for harnessing the heat energy. In a fusion reactor, by contrast, any such interface would disrupt the plasma and stop the fusion reaction. ITER’s design will demonstrate heat removal, but more R&D is needed to raise the efficiency for practical electricity generation.
With these challenges as context, we can now come to a better—if still imperfect—answer to the question. Does nuclear fusion have the potential to be a sustainable energy solution?
Clearly, the answer is yes. Fusion holds incredible potential for energy production. The more critical question is when? and what will it take? The near-term potential for fusion commercialization, in our view, remains low; it would require multiple extraordinary breakthroughs. The medium- to long-term potential is much more positive. The challenges of ITER have demonstrated many of the hurdles to be overcome, but we are making strong progress. In parallel, the private sector is beginning to contribute meaningfully, often using concepts and designs which—although less tested and involving higher risk—may in some cases address fusion’s challenges in new, innovative ways.
These public and private sector efforts are complementary. The more we work together, with appropriate funding and shared solutions, the higher the potential, and—possibly—the faster we can bring fusion to reality.
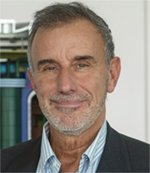
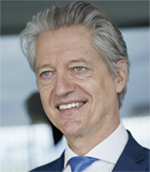